Our research team has developed computational fluid dynamics and heat transfer models of waste glass melters in support of the vitrification campaign at the Waste Treatment and Immobilization Plant (WTP) as part of the United States nuclear waste stewardship and environmental protection programs. The WTP melters are being constructed to process legacy nuclear waste into a stable form for disposal. This research strives to ensure that the vitrified waste will be stable, durable, and processed in the safest, most efficient and economical manner possible. Hanford’s tank waste is far more complex and varied than the waste treated by other vitrification facilities and both startup and long-term operational challenges are likely to be even greater than prior experience has shown. There are approximately 55,000 different waste compositions that make processing the waste a complex and challenging task. The best capabilities throughout the world have been harnessed and a team of experts has been assembled to apply their knowledge and experience to this legacy cleanup problem. The results of these modeling activities will be used to support startup to hot operations at the WTP.
Computational modeling is a powerful complement to experimental testing to provide insights into the complex physicochemical phenomena occurring inside the waste glass melters. The radioactivity, high temperature, opaqueness of the molten glass, and surrounding structure will make it difficult to troubleshoot the melters after they are commissioned and fully operational. Idaho National Laboratory has led the effort to model glass furnaces of various sizes spanning from laboratory crucibles, to pilot-scale melters, to full-scale WTP low-activity waste (LAW) and high-level waste (HLW) melters. Additionally, models have been developed to investigate specific phenomena occurring in the melters, such as pneumatic mixing by melt bubbling to induce forced convection, foaming underneath the cold cap, Joule heating from the electrodes, plenum heat transfer, off-gas generation, refractory corrosion, cold cap motion, and batch-to-glass conversion kinetic rates. Validation data were obtained from pilot-scale tests conducted at the Vitreous State Laboratory at the Catholic University of America and laboratory experiments at Pacific Northwest National Laboratory (PNNL), the University of Chemistry and Technology (UCT) Prague, and Savannah River National Laboratory (SRNL).
The commercial computational fluid dynamics (CFD) modeling software STAR-CCM+ is the base platform used to perform simulations. STAR-CCM+ uses the Eulerian multiphase volume of fluid (VOF) approach and the Reynolds Averaged Navier-Stokes equations to model two-phase flow. The solution methodology is based on a finite volume approach with first-order implicit time-stepping and second-order spatial discretization. The segregated flow solver for the Navier-Stokes equations is used, which can handle constant density or mildly compressible flows with a predictor-corrector approach coupling the momentum and continuity equations. A collocated variable arrangement and a Rhie-and-Chow type pressure-velocity coupling are combined with a SIMPLE-type algorithm. Due to the low Reynolds number (~30), the laminar model is used. In most models, bubbling air and foaming gases are represented as single-component gas phases; but some sets of models have been run to distinguish the major gas species for oxygen, nitrogen, water vapor, and carbon dioxide. Mesh convergence on a set of coarse, medium, and fine meshes is assessed using a grid convergence index across all melter sizes.
High-fidelity simulations of forced convection bubbling require the use of a modeling framework capable of fully resolving the free surface at the glass/air interface affected by large, spherical-cap-shaped bubbles produced by orifices located at the base of the melter. STAR-CCM+ uses the VOF method whereby volume fraction equations are solved for different immiscible phases of the system. Simplified models were constructed to reduce the required computational time to a manageable amount. A momentum source term approach has been implemented by necessity in representations that include a more complete description of the melter construction, which includes the refractory and insulation layers, for studying large timescale changes in the temperature for operational step changes or potential failure modes.
The cold cap is modeled using conjugate heat transfer into a rigid solid. Cold cap submodels have been developed based on evolved gas analysis and foam expansion test results conducted at PNNL and UCT Prague for various feed compositions. These submodels are integrated into the overall melter model using fitted polynomial equations of response surfaces. The effect of cold cap motion on heat transfer is being investigated using a dynamic fluid-body interaction submodel that has been validated using experiments in a room temperature, transparent vessel at SRNL. As opposed to a rigid cold cap approximation, the transparent melter experiments are conducted with a 3D printed, flexible material to mimic the cold cap. Machine learning has also been applied to determine the cold cap configuration based on plenum temperatures measured by thermocouples. Air inleakage through the refractory bricks and penetrations has been accounted for using a heat sink term. This information can help operators determine recovery strategies for anomalies or off-normal events.
CFD has been used to understand the effect of cavity movement beneath the cold cap on the batch melting rate both with and without bubbling induced by flowing gas into the melt to promote forced convection. The cavities beneath the cold cap are a result of collapsing primary foam, ascending secondary foam, and bubbling. Simulations for a continuous laboratory scale melter were performed for three gas evolution rates and three cold cap shapes – a solid disk, a disk with a central vent hole, and a disk touching the wall with a central vent hole. Ongoing research is examining the formation and behavior of the cavity layer using in-situ X-Ray computed tomography.
Refractory corrosion has been assessed through thermodynamics modeling and crucible tests exposing the refractory coupons for various durations. Numerical models are being developed to predict corrosion rates and extrapolate melter refractory lifetime to operational melters based on the observed phenomena. This combined experimental-computational approach seeks to overcome the shortcomings of scaled crucible tests in relation to the complex dynamics in operational melters.
The plenum is filled with a large volume of gases generated from the batch reactions. The major gaseous emissions released from the melter are H2O, N2, O2, and CO2 along with various monitored environmental pollutants, such as NO, N2O, NO2, or SO2. In addition, reactions between organics and nitrates in the feed may also form products of incomplete combustion such as carbon monoxide (CO) and acetonitrile (CH3CN). Predictive modeling using both analytical regression-based and machine learning approaches has been applied to determine the gas composition generated by various feeds. This approach can significantly reduce the amount of expensive physical melter testing and assist with the optimization of feed composition with respect to off-gas composition.
Recently, we have been developing a model to use the open source Nek5000 code to provide faster turnaround. This platform uses the spectral element method, which is efficient and scalable. Techniques for Joule heating and two-phase flow are being incorporated. With further development, the NekRS version of the code (which is deployable on GPUs), offers the potential to further increase the turnaround time of numerical simulations to support WTP operations.
The current modeling capability has been developed over the last decade in preparation for melter startup before the end of 2023. The least radioactive of the tank waste (i.e., LAW), which accounts for roughly 90% by volume of the total tank waste, will be treated first. The commissioning process is underway for the two 300-ton LAW melters. The first melter will be heated up, commissioned and verified operational before the second melter is heated and tuned. HLW will be vitrified in a separate facility.
As a result of advances in the understanding of waste processing and underlying glass science for vitrification over the last decade, the Agency is pursuing the concept of direct feed HLW. Without the need for an additional facility at the WTP for the separation of the LAW fraction, there exists the opportunity to realize an economic and schedule opportunity to start treatment of the HLW fraction much sooner.
Abstract
Numerical modeling of Joule Heated Ceramic Melter
Numerical modeling of Joule Heated Ceramic Melter
Donna Post Guillen 1, Alexander W. Abboud 1, Pavel Ferkl 2, Richard Pokorny 3,4, Mark Hall 2, Pavel Hrma 5, Albert A. Kruger 6
1 Idaho National Laboratory, Idaho Falls, ID 83415, United States of America
2 Pacific Northwest National Laboratory, Richland, WA 99354, United States of America
3 Laboratory of Inorganic Materials, University of Chemistry and Technology Prague, Prague 6, 166 28, Czech Republic
4 Institute of Rock Structure and Mechanics, Czech Academy of Sciences, Prague 8, 182 09, Czech Republic
5 AttainX, Support Services Contractor to the Office of River Protection, U.S. Department of Energy, Richland, WA 99354, United States of America
6 U.S. Department of Energy, Office of River Protection, Richland, WA 99354, United States of America
2 Pacific Northwest National Laboratory, Richland, WA 99354, United States of America
3 Laboratory of Inorganic Materials, University of Chemistry and Technology Prague, Prague 6, 166 28, Czech Republic
4 Institute of Rock Structure and Mechanics, Czech Academy of Sciences, Prague 8, 182 09, Czech Republic
5 AttainX, Support Services Contractor to the Office of River Protection, U.S. Department of Energy, Richland, WA 99354, United States of America
6 U.S. Department of Energy, Office of River Protection, Richland, WA 99354, United States of America
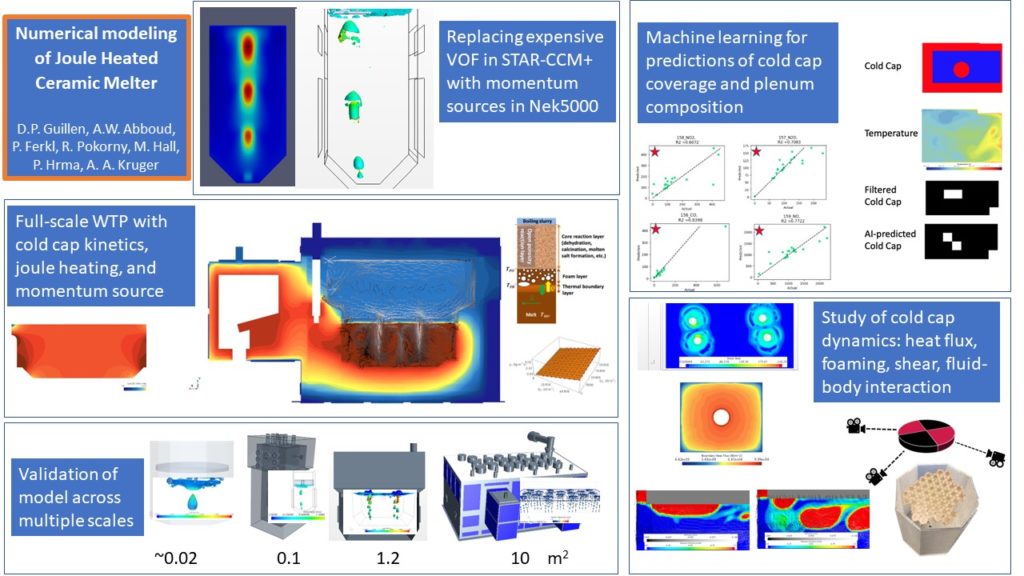
- Type: Guest oral presentation
- Related categories: Vitrification process/technologies